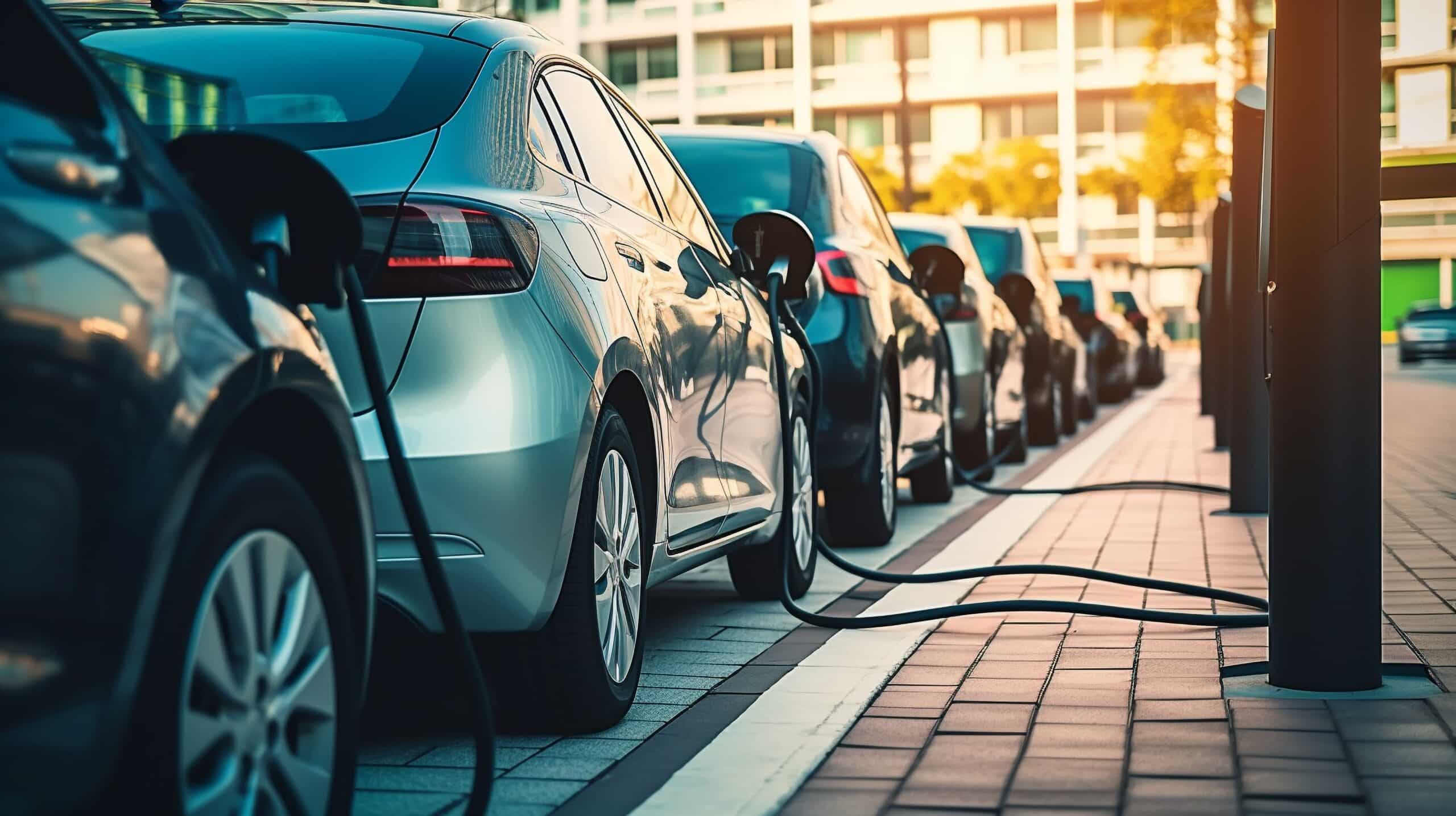
ELECTRIC VEHICLES: THE SLOW ADOPTION SYNDROME
In 1779, Ned Ludd, a young apprentice in England working in the textile industry, destroyed a mechanized loom after his supervisor scolded him for not managing the machine well. Thus were born the Luddites, a movement that fiercely, sometimes violently opposed the introduction of new machinery during the industrial revolution, on the grounds that it would displace workers and bring about a major social disruption. It took military intervention to suppress the Luddite movement but, after some delay, the industrial revolution became an unstoppable force that transformed the British economy, and later economies around the world. It was not the first time that the emergence of a new technology caused fear, anxiety, and sometimes violence, and it wouldn’t be the last.
Other factors have also contributed to the slower adoption of technologies that, later, would prove to be overwhelmingly superior to those they were replacing. It took decades after the invention of the incandescent light bulb by Thomas Edison in 1879 before electricity became commercially viable. Many obstacles initially stood in the way: poor technological development of the early electrical systems; lack of infrastructure; high investment without a clear return; simple lack of awareness and acceptance by the public; and the lack of an appropriate regulatory framework that promoted standardized practices. Something similar happened with the introduction of Tractors. While the word “tractor” started to be used only in the early 20th century, they had been in use in Britain and the US since at least 1890. However, by 1920 only about 4% of American farms had a tractor, and by 1940 the number had gone up to just 23% (interestingly, a higher percentage of farmers had cars). Only after the end of the war did the tractor become a symbol of American farming. What accounts for the slow diffusion? Again, initially the technology was poor; tractors remained big and rudimentary until at least the late 1920s. Secondly, wages remained low, and the combination of horse and manpower proved more competitive during the years of the depression and up to the end of the war. And thirdly, it was hard to justify making the investment in a tractor in a small farm given the upfront investment. The transformation of agriculture into large farms was necessary to enable the massification of tractors on a commercial scale.
Is this “slow adoption syndrome” also affecting the transition to electric vehicles (EVs)? After the initial boom in EV sales, powered by Government subsidies in China, regulations in Europe and the passage of the IRA in the US Congress in 2022, global sales of EVs have started to show signs of a slowdown, particularly in Europe and the US, and continue to power ahead only in China. While sales of “electrified” vehicles in the US accounted for 20% of the total in 2024, of the 3.2 million units sold 1.9 million were hybrids, including plug-in models, and only 1.3 million were all-electric EVs. If, as expected, the new US administration succeeds in removing the -up-to- $7,500 subsidy on purchases of EV models, sales of EVs would still grow modestly, to perhaps 10% of the total in 2025, or 25% of the total if hybrid vehicles are included. Were that projection to materialize, this would be the fourth consecutive year of growth in the share of EVs, but it would still fall way short of the ambitious targets set by the OEMs and the Biden administration four years ago. More importantly, it would make the forecast of 30% of total sales by 2030 a distant prospect, and the target of carbon neutrality by 2050 even harder to achieve. This article, the first in a three-part series on the reasons behind the slower adoption of EVs, will focus on the state of the technology on batteries, by far the main component of the cost (about 40% of the total), as well as the most important factor in the relative appeal of EV models compared with traditional ICE vehicles for consumers.
In lithium-ion batteries, the most common technology, when the battery is charging electrons are stripped from lithium atoms on the cathode to create charged particles called ions. These then migrate through an electrolyte to the anode. When the battery discharges the process reverses and the electrons in the circuit power the electric motor. The most common chemistry in the cathode is a combination of Nickel, Manganese and Cobalt (NMC). NMC batteries are highly efficient from an energy density standpoint, but Cobalt and Nickel are expensive, relatively scarce and, in the case of Cobalt, mining is concentrated in the Democratic Republic of Congo, where illegal mining is rampant, conditions are dangerous, and child labor is widely used. Lithium is the lightest metal, stores more energy per unit of weight, possesses fast charging capabilities, and has proven to be long-lasting compared with other metals. However, lithium is also relatively scarce and expensive, its reserves are concentrated in Argentina, Australia and Chile, and extraction faces resistance from communities and public opinion because of the environmental impact in lithium carbonate form for use in batteries. Even in a slow-growth environment for EVs, demand for lithium is expected to rise to at least 2.4 million tons by 2030, from about one million tons today. The refining of these minerals is dominated by China, where 90% of global processing of battery cells is currently done. China refines almost all the world’s manganese and over 70% of lithium. Anodes in lithium-ion batteries primarily use graphite for its safety, stability, conductivity and relative cost competitiveness (especially in synthetic form). However, graphite-based anodes offer low energy density, shorter range and longer charging times, and China also controls most of its processing.
With all the limitations and geopolitical risks of the current lithium-ion batteries, it is no wonder that a furious race is under way to replace or reduce the use of the current metals and change the chemistry in batteries to improve efficiency and range, reduce cost, shorten charging times and shake China’s stranglehold on the refining of minerals. A combination of advances in battery recycling and different battery chemistries promises to eliminate the need for minerals extraction by 2050. Under this scenario, demand for raw materials used in batteries may peak by the mid-2030s, with more than 50% of minerals coming from recycled batteries. Currently, battery recycling is confined to the recovery of minerals from the scrap produced at the gigafactories. The reason is that traditional battery recycling is labor and energy intensive and poses major environmental challenges. However, through a process called pyrometallurgy, where batteries are “cooked” at very high temperatures, nickel and cobalt can now be recovered far more efficiently with less hazard. Recovering lithium is harder, but hydrometallurgy, a process where batteries are crushed and treated with chemicals, offers good prospects. Graphite has proven more intractable, as the need to purify it before it can be used in anodes makes recovery from an old battery exceedingly difficult. However, two firms in Australia and Sweden claim to have made significant strides in “re-purifying” graphite from scrap and old batteries, through a chemical purification process of which little is yet known, at least publicly.
Finding good alternative chemistries to NMC batteries has not been an easy task. Manganese and cobalt are good at stabilizing and boosting performance in lithium-ion batteries, while nickel helps increase energy density, which means more energy can be stored in a smaller, lighter battery. In this area, Lithium-iron phosphate (LFP) batteries have found good acceptance. LFPs don’t need nickel and cobalt, but they deliver lower energy density and are thus used primarily for short-distance urban use in China, though some Tesla models in the US also run on LFP batteries. Sodium is being tested as an alternative to lithium. In addition to being relatively cheap and abundant, it can form cathodes with manganese, iron and other inexpensive metals. Its main drawback is weight, which means it is proving to be a good alternative for heavy trucks and energy storage, where battery weight is less important. CATL, the largest battery manufacturer in the world, and other Chinese battery companies are already building factories for sodium batteries. Research is also under way to find alternatives to graphite as the base for anodes. The most promising is the use of silicon (or a silicon-carbon composite). Silicon is plentiful and can absorb far more lithium ions than graphite, which substantially improves performance by increasing energy density. The catch is that it tends to swell and can damage the battery in the process. Group 14 Technologies, a company in Seattle, has started to develop anodes using silicon.
In addition to LFP batteries, which have gained wide acceptance for small vehicles, solid state batteries, where a solid electrolyte made of ceramic or glass is used instead of the liquid or gel found in lithium-ion batteries, appear close to being launched on a commercial scale. They provide higher energy density, improved safety, faster charging, and potentially a longer life span. They also need substantially less cobalt and nickel than traditional batteries. Notwithstanding their clear advantages, solid state batteries present significant manufacturing challenges for production in large volumes, due to the type of equipment necessary and the manufacturing techniques involved. Not surprisingly, among the OEMs Toyota, which retains a significant lead in manufacturing over its main competitors, seems closest to using solid state batteries for large volume models. At a recent press conference, Koji Sato, the CEO of the company, announced that Toyota will introduce solid state batteries in high volumes by 2027.
In short, the race is on to develop battery cells that are safer, lower cost, deliver higher energy density per unit of weight, and offer significantly more range and shorter charging times. While the technical challenges remain high, significant strides have been made on all fronts, and it is not unreasonable to assume that before the end of the decade EVs will be profitable for the car manufacturers, competitive with ICE vehicles, and provide enough appeal to customers to be the preferred technology in the market. All this, despite the political headwinds in the short term. Achieving these milestones will require a 50% cost reduction from the current average of $110 KW/Hr. This is a prospect that no longer seems outlandish.
In the next article, I will review progress in the development of a charging infrastructure, the other essential leg in the electrification of transportation.
Jaime Ardila
The Hawksbill Group